Exoplanet host stars
The observational study of extrasolar planetary systems is a very recent topic. Instruments capable of detecting the radial-velocity variations of a solar-like star induced by orbiting Jupiter-like planets were built in the 1970s. These led to the detection of the first extrasolar planet in 1995, a hot Jupiter in a very close orbit around the star 51 Peg. During the following years, the radial velocity method was the most successful method for planet discovery, with steadily increasing detection numbers. Until 2002, 60 planets had been detected, and from 2002 to 2010, about 30 to 80 planets per year were detected.
More recently, a large number of extrasolar planets have been detected with the transit method, by ground-based telescope networks such as SuperWASP or HATNet, and by the space missions CoRoT and Kepler. When a planet passes in front of the star as seen from the Earth, a small decrease in the stellar brightness is measured. The relative change in brightness (the deepness of the dip in the light curve) is equal to the ratio of the projected areas of the planet and the star – between about 0.01% and 1%. However, a similar change in brightness can be caused by other phenomena, e.g. stellar variability or grazing eclipsing binary stars. Any transit detection needs to be confirmed with radial velocity measurements.
In total, more than 4000 planets around more than 3000 stars are known so far (2019-09-24, http://exoplanet.eu/catalog/). On-line databases such as http://exoplanet.eu or https://exoplanetarchive.ipac.caltech.edu/ can be consulted for updated numbers. The radial-velocity data and transit light-curves are used together with known properties of the host stars to infer planet properties, such as mass and radius. The planet and star properties can also be found in the on-line databases. Comparing the planet properties to models of planetary interiors gives us clues on what type of planet has been detected – a small rocky one similar to the Earth, an icy planet like Uranus, or a large gas planet like Jupiter.
Furthermore, stellar properties such as surface temperature and size determine the region around a star in which water can remain in its liquid state. This is regarded as a prerequisite for life and the region is therefore called the “habitable zone” (see Fig. 1). Most of the currently known planet hosts have properties resembling those of the Sun – simply because most planet searches chose solar-like stars as their targets. More specifically, the masses of 95% of planet hosts are within a factor two of the solar mass, and the remaining stars are in equal parts more or less massive. The distribution of surface gravities shows that almost 90% of the host stars are dwarf stars, and half of the remaining stars are in roughly equal parts subgiants or giant stars. Almost 60% of the host stars have surface temperatures between 5500 and 6500 K, 40% below 5500 K, and just 3% or 30 stars are M dwarfs with temperatures below 4000 K.
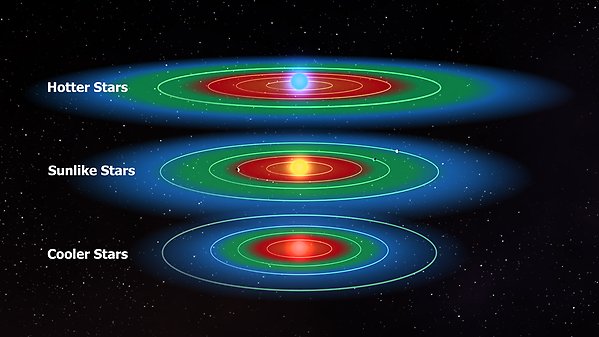
Fig. 1: Schematic of habitable zones around different stars. The green region is at the proper distance from the star where water can remain in its liquid state on the surface of a planet. The red region is too warm, and the blue region is too cool. Image: NASA/Kepler Mission/Dana Berry.
Already a few years after the first planet detection, spectroscopic studies of the host stars indicated that most of them had a higher content of heavy elements like iron than the Sun (a higher metallicity). This was later confirmed by several research groups (among others Heiter & Luck 2003, The Astronomical Journal 126, 2015). This planet-metallicity correlation was quantified by Fischer & Valenti (2005, The Astrophysical Journal 622, 1102). From a metallicity comparison for stars with and without Saturn- to Jupiter-mass planets they concluded that the probability of forming a gas giant planet is nearly proportional to the square of the number of iron atoms. Also, all other chemical elements that can be measured in solar-like stars showed an equally enhanced abundance in planet hosts relative to comparison stars (Fig. 2). This indicates that the explanation for the planet-metallicity correlation is given by the intrinsically higher metallicity of the molecular clouds from which the majority of the planetary systems have formed (and not by some kind of “pollution” or other surface phenomenon appearing after star formation).
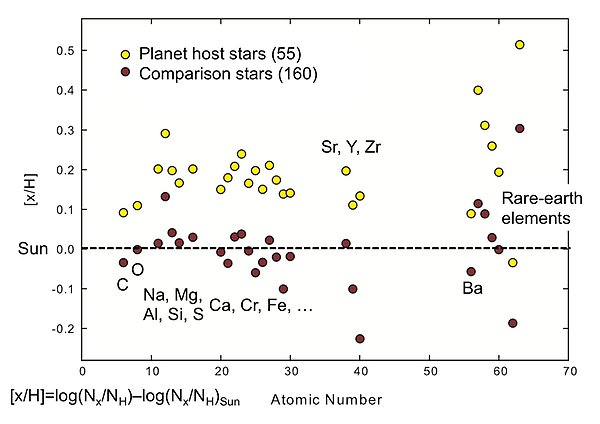
Fig. 2: The abundances of chemical elements in planet host stars. The logarithmic abundance scale [x/H] is defined as the logarithm of the number abundance of element x relative to hydrogen measured for the star minus the same quantity measured for the Sun. Figure: adapted from Luck & Heiter (2006, AJ 131, 3069).
More recent studies have investigated the metallicities of solar-like stars hosting lower-mass and smaller planets (4 to 25 Earth masses or 1 to 4 Earth radii), and found distributions centred around solar metallicity. Thus it seems that metallicity plays a key role for planet formation around solar-like stars. For the smaller and cooler host stars, the M dwarfs, the situation is less clear due to the currently smaller sample size and more difficult metallicity determination. These stars are however very interesting as the probability to find small planets and planets within the habitable zone is higher around M dwarfs than solar-like dwarfs. In Uppsala, we are developing methods for accurate metallicity determination and abundance analysis of M dwarfs, based primarily on high-resolution spectroscopy in the infrared wavelength region.
Magnetic fields of the host stars and planets represent an important factor that determines characteristics of the planetary atmospheres and habitability of exoplanets. Magnetic activity on stellar surfaces is associated with energetic phenomena, such as flares, short-wavelength emission, powerful stellar winds. This creates a hostile environment in which close-in rocky exoplanets may completely loose their atmospheres. On the other hand, moderate amounts of UV radiation, similar to the levels exhibited by the young Sun, contribute to the photochemical reactions in planetary atmospheres resulting in formation of complex organic molecules. Furthermore, a strong enough planetary magnetic field can protect it from damaging effects of stellar magnetic activity, providing favourable conditions for the development of life.
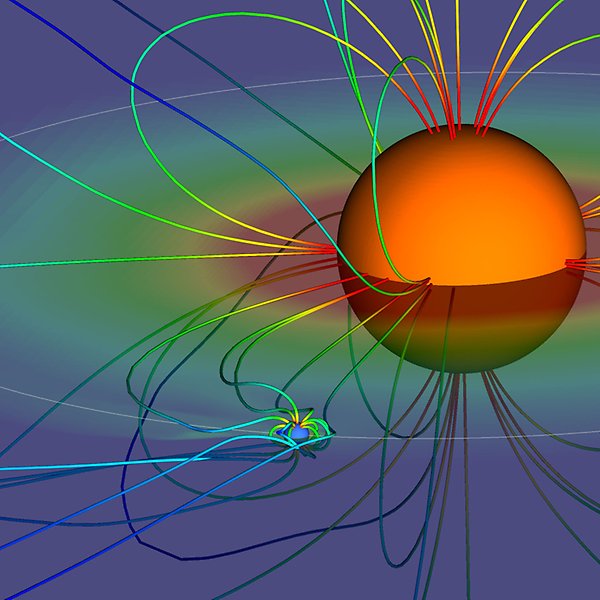
Computer simulation showing magnetic interaction between a star and its nearby planet. Image: A. Strugarek, Université de Montréal
In Uppsala we pursue a systematic investigation of magnetic properties of exoplanet host stars. We determine the strength and topologies of stellar surface magnetic fields using spectropolarimetric observations. This information is then used to deduce an extended 3-D structure of stellar magnetospheres and to study in detail the magnetic interactions between planets and their host stars. These studies represent the only known way of estimating the strength of planetary magnetic fields. A related direction of our research is investigation of the magnetic field properties of young solar analogues. These stars, being similar to the Sun at the time when the life has emerged on Earth, represent unique natural laboratories for assessment of the conditions that existed on Earth billions of years ago.
In summary
We need to determine the properties of planet host stars with high precision
- in order to determine precise planet properties (radii and masses, giving planet type),
- to determine the location of the habitable zone (depending on stellar temperature and radius),
- to characterise magnetic interaction between the stars and planets and assess impact of the stellar activity on exoplanet atmospheres
- and to constrain the favourable conditions for planet formation, in particular the metal content of the parent molecular cloud.
Contact
Ulrike Heiter, Bengt Edvardsson, Nikolai Piskunov, Eric Stempels, Oleg Kochukhov, Terese Olander