Condensed Matter Physics of Energy Materials

We characterize functional materials at an atomic level by a variety of advanced element specific and time-resolved X-ray based spectroscopies. Our aim is to connect various functional materials aspects with specific atomic species, chemical environments, surface and bulk properties, dimensionality and time-response scale.
These fundamental investigations will enable us to design materials of desirable properties useful in for example magnetism and energy applications. These complex materials also represent an opportunity to advance the development of spectroscopy.
Condensed matter properties that relate to the intrinsic spin of electrons have become increasingly more studied in the last few decades. This can be connected to the success of magnetoresistance effects that resulted in significant technological advances and due to novel spin phenomena that show potential for future applications. At the division of X-ray Photon Science, we study the fundamental properties of magnetic materials using mainly photon and transport based methods. The aim is to develop the understanding of the dynamic magnetic properties, electronic structure, and spin transport in novel materials.
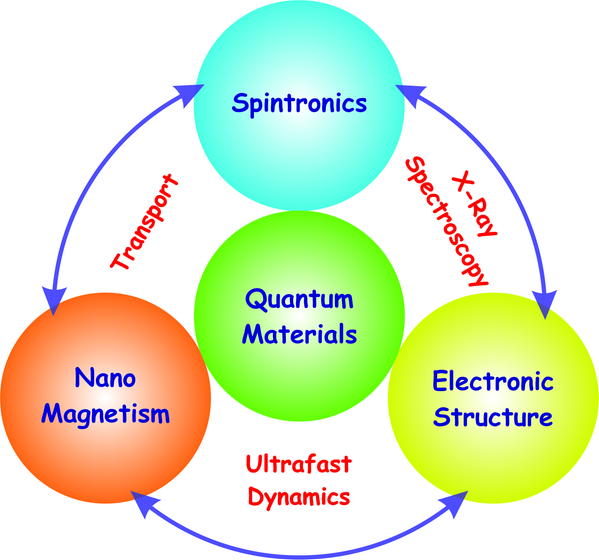
Our research is described in the following subsections:
The research is performed both at synchrotrons and at the following local laboratories:
Magnetism - Spintronics and dynamics
Our investigations on modern magnetism and spintronics focus on understanding and controlling atomic spins in space and time. We study how the behavior of atomic spins depend on the sample structure/composition and how they are affected by various types of excitations. With this we aim to contribute to the development of spintronic components for information technology, that for instance can lead to faster and more energy efficient memories. The techniques include X-ray magnetic circular dichroism (XMCD), X-ray photoelectron spectroscopy (XPS), X-ray ferromagnetic resonance (X-FMR) and time-resolved transverse magneto-optic Kerr effect (T-MOKE) with IR pump and EUV probe.
Energy Materials – Conversion and storage
One of the grand challenges facing humankind is connected to energy. We aim to develop a fundamental understanding of key processes on the atomic level connected energy conversion. Much of the research concerns investigations on complex interfaces and its dynamics. We specifically focus on the conversion between solar energy, electrical energy and chemical energy in applications such as solar cells, batteries and catalysis/photocatalysis.

Energy Materials
Energy Materials – Surfaces and interfaces for conversion and storage
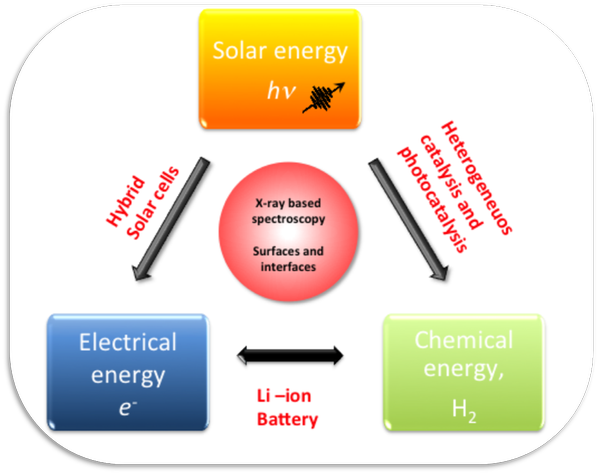
One of the great challenges of our modern society is to meet the growing energy demand by using sustainable, efficient and renewable energy sources. At the division of X-ray Photon Science, the research is partly based on fundamental understanding of electronic structure and electron dynamics in solid and molecular materials. We specifically study the atomic structure of various materials used in energy applications and relate this structure to their functionality in the device.
Our research on electronic structure and electron dynamics is subdivided into the following interlinked areas:
The use and development of X-ray based spectroscopy tools is an integrated part of the work with the aim of solving key questions for an improved understanding of the working principle of the energy materials. This will contribute to the development of better and more efficient applications.
Projects: We invite students interested in doing a Master and Bachelor project. For more details about available projects in different research areas field please contact:
Fundamentals: Andreas Lindblad
Photovoltaics: Bertrand Philippe
Batteries: Maria Hahlin
Catalysis/Photocatalysis: Laurent Duda
Fundamentals of Energy Materials
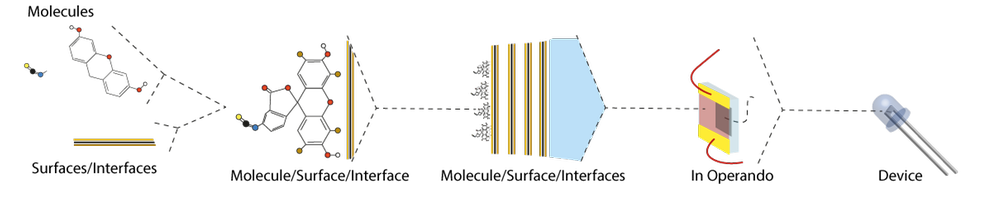
Many fundamental processes, e.g. for energy conversion, occur at the interfaces between different materials and phases. The interface structure often differs from the pure three-dimensional structure, and these differences affect materials electron structure and function.
Within our group we study the basic structure of surfaces and interface layers between pure solid materials. These kind of investigations are a crucial requirement to understand, control and tune the electronic and optical properties of functional materials for improving the performance of technological devices (solar cells, batteries, OLED, charge-coupled devices, field effect transistors and others). Furthermore, we study novel molecular materials starting with the characterization of the free molecular building blocks and ending with investigations of the more complex, assembled molecule–surface systems and complex nanostructures. Special focus is put on the modifications induced on the different parts of the system due to their functionalization or interaction with the substrate.
Our research extends between more well-defined model systems to more real and complex systems. In particular, we study electron dynamics on different time scales (from attoseconds to nanoseconds) and how molecules interact with surfaces in different environments. In this way the research area combines knowledge of single molecules and three-dimensional solid materials.
To address such a broad range of the research questions we use a rich set of X-ray spectroscopy techniques including XPS, ARPES, time resolved-PES, NEXAFS and obtain complementary information using the microscopy tools (for example STM, AFM, LEEM, PEEM). We perform our experiments both at synchrotron radiation facilities and by using infrastructure at the Ångström Laboratory (e.g. HELIOS).
People
- Andreas Lindblad (assistant professor)
- Carla Puglia (associate professor)
- Anders Sandell (professor)
- Konstantin Simonov (researcher)
- Fredrik Johansson (PhD student)
- Teng Zhang (PhD student)
- Håkan Rensmo (professor)
Selected papers
Effect of Substrate Chemistry on the Bottom-Up Fabrication of Graphene Nanoribbons: Combined Core-Level Spectroscopy and STM Study
K. A. Simonov, N. A. Vinogradov, A. S. Vinogradov, A. V. Generalov, E. M. Zagrebina, N. Mårtensson, A. A. Cafolla, T. Carpy, J. P. Cunniffe, and A. B. Preobrajenski
J. Phys. Chem. C 118, 12532-12540 (2014)
From Graphene Nanoribbons on Cu(111) to Nanographene on Cu(110): Critical Role of Substrate Structure in the Bottom-Up Fabrication Strategy
K. A. Simonov, N. A. Vinogradov, A. S. Vinogradov, A. V. Generalov, E. M. Zagrebina, G. I. Svirskiy, A. A. Cafolla, T. Carpy, J. P. Cunniffe, T. Taketsugu, A. Lyalin, N. Mårtensson, and A. B. Preobrajenski
ACS Nano 9, 8997-9011 (2015)
Minimizing sputter-induced damage during deposition of WS2 onto graphene
FOL Johansson, P Ahlberg, U Jansson, SL Zhang, A Lindblad, T Nyberg
Applied Physics Letters 110 (9), 091601 (2017)
Two dimensional band structure mapping of organic single crystals using the new generation electron energy analyzer ARTOF
A Vollmer, R Ovsyannikov, M Gorgoi, S Krause, M Oehzelt, Andreas Lindblad, Nils Mårtensson, Svante Svensson, P Karlsson, M Lundvuist, T Schmeiler, J Pflaum, N Koch
Journal of Electron Spectroscopy and Related Phenomena 185 (3-4), 55-60 (2012)
Elucidating the 3d Electronic Configuration in Manganese Phthalocyanine
Iulia E. Brumboiu, R. Totani, M. de Simone, M. Coreno, C. Grazioli, L. Lozzi, Heike C. Herper, B. Sanyal, O. Eriksson, C. Puglia and B. Brena
J. Phys. Chem. A 118, 927 (2014)
The electronic characterization of biphenylene – experimental and theoretical insights from core and valence level spectroscopy
J. Lüder, M. De Simone, R. Totani, M. Coreno, C. Grazioli, B. Sanyal, O. Eriksson, B. Brena, and C. Puglia
J. Chem. Physics, 142, 74305 (2015)
The formation of the wetting layer on TiO2: A molecular mechanism for the water-hydroxyl balance
M. Amft, L. E. Walle, D. Ragazzon, A. Borg, P. Uvdal, N. V. Skorodumova, and A. Sandell
J. Phys. Chem. C 117, 17078 (2013)
Research links
We are partners in the Uppsala-Berlin joint Laboratory (UBjL) at HZB/BESSY and have partaken in the build-up, commissioning and currently engage in further development of the infrastructure of the LowDosePES and CoESCA beamlines there. At UU we are partners in the LaMeS-program (associated with the Graphene Flagship) on research of layered metal sulphides. At MAX IV we are engaged in the VERITAS beamline and in the DiffMAX-project.
Master and Bachelor projects
We invite students interested in master and bachelor projects. For more details about available projects, please contact Andreas Lindblad.
Many fundamental processes, e.g. for energy conversion, occur at the interfaces between different materials and phases. The interface structure often differs from the pure three-dimensional structure, and these differences affect materials electron structure and function.
Within our group we study the basic structure of surfaces and interface layers between pure solid materials. These kind of investigations are a crucial requirement to understand, control and tune the electronic and optical properties of functional materials for improving the performance of technological devices (solar cells, batteries, OLED, charge-coupled devices, field effect transistors and others). Furthermore, we study novel molecular materials starting with the characterization of the free molecular building blocks and ending with investigations of the more complex, assembled molecule–surface systems and complex nanostructures. Special focus is put on the modifications induced on the different parts of the system due to their functionalization or interaction with the substrate.
Our research extends between more well-defined model systems to more real and complex systems. In particular, we study electron dynamics on different time scales (from attoseconds to nanoseconds) and how molecules interact with surfaces in different environments. In this way the research area combines knowledge of single molecules and three-dimensional solid materials.
To address such a broad range of the research questions we use a rich set of X-ray spectroscopy techniques including XPS, ARPES, time resolved-PES, NEXAFS and obtain complementary information using the microscopy tools (for example STM, AFM, LEEM, PEEM). We perform our experiments both at synchrotron radiation facilities and by using infrastructure at the Ångström Laboratory (e.g. HELIOS).
People
- Andreas Lindblad (assistant professor)
- Carla Puglia (associate professor)
- Anders Sandell (professor)
- Konstantin Simonov (researcher)
- Fredrik Johansson (PhD student)
- Teng Zhang (PhD student)
- Håkan Rensmo (professor)
Selected papers
Effect of Substrate Chemistry on the Bottom-Up Fabrication of Graphene Nanoribbons: Combined Core-Level Spectroscopy and STM Study
K. A. Simonov, N. A. Vinogradov, A. S. Vinogradov, A. V. Generalov, E. M. Zagrebina, N. Mårtensson, A. A. Cafolla, T. Carpy, J. P. Cunniffe, and A. B. Preobrajenski
J. Phys. Chem. C 118, 12532-12540 (2014)
From Graphene Nanoribbons on Cu(111) to Nanographene on Cu(110): Critical Role of Substrate Structure in the Bottom-Up Fabrication Strategy
K. A. Simonov, N. A. Vinogradov, A. S. Vinogradov, A. V. Generalov, E. M. Zagrebina, G. I. Svirskiy, A. A. Cafolla, T. Carpy, J. P. Cunniffe, T. Taketsugu, A. Lyalin, N. Mårtensson, and A. B. Preobrajenski
ACS Nano 9, 8997-9011 (2015)
Minimizing sputter-induced damage during deposition of WS2 onto graphene
FOL Johansson, P Ahlberg, U Jansson, SL Zhang, A Lindblad, T Nyberg
Applied Physics Letters 110 (9), 091601 (2017)
Two dimensional band structure mapping of organic single crystals using the new generation electron energy analyzer ARTOF
A Vollmer, R Ovsyannikov, M Gorgoi, S Krause, M Oehzelt, Andreas Lindblad, Nils Mårtensson, Svante Svensson, P Karlsson, M Lundvuist, T Schmeiler, J Pflaum, N Koch
Journal of Electron Spectroscopy and Related Phenomena 185 (3-4), 55-60 (2012)
Elucidating the 3d Electronic Configuration in Manganese Phthalocyanine
Iulia E. Brumboiu, R. Totani, M. de Simone, M. Coreno, C. Grazioli, L. Lozzi, Heike C. Herper, B. Sanyal, O. Eriksson, C. Puglia and B. Brena
J. Phys. Chem. A 118, 927 (2014)
The electronic characterization of biphenylene – experimental and theoretical insights from core and valence level spectroscopy
J. Lüder, M. De Simone, R. Totani, M. Coreno, C. Grazioli, B. Sanyal, O. Eriksson, B. Brena, and C. Puglia
J. Chem. Physics, 142, 74305 (2015)
The formation of the wetting layer on TiO2: A molecular mechanism for the water-hydroxyl balance
M. Amft, L. E. Walle, D. Ragazzon, A. Borg, P. Uvdal, N. V. Skorodumova, and A. Sandell
J. Phys. Chem. C 117, 17078 (2013)
Research links
We are partners in the Uppsala-Berlin joint Laboratory (UBjL) at HZB/BESSY and have partaken in the build-up, commissioning and currently engage in further development of the infrastructure of the LowDosePES and CoESCA beamlines there. At UU we are partners in the LaMeS-program (associated with the Graphene Flagship) on research of layered metal sulphides. At MAX IV we are engaged in the VERITAS beamline and in the DiffMAX-project.
Master and Bachelor projects
We invite students interested in master and bachelor projects. For more details about available projects, please contact Andreas Lindblad.
Photovoltaics

With the help of solar cells, solar energy can be directly converted to electrical energy with minimal environmental impact. Common to all solar structures is an interface layer where the sunlight is absorbed by exciting electrons (e-) and where the electrons are then rapidly transported from their positive environment, e.g. hole (h+). Through this process, the electrons increase their potential energy and create a photovoltage (U) which can then be used for an electrical current (I) and taken out as electrical energy with the power P = U x I.
The research focuses on understanding the formation and character of electrons and holes as well as how the charge separation takes place at the atomic level. From this basic understanding, we learn how to build new materials and interfaces that effectively and with high stability can be used to make the conversion from the sun's light to electricity as efficient and sustainable as possible.
Our activity focuses on the investigation of emerging solar cell technologies such as Perovskite Solar Cells (PSC), Quantum Dots Cells or Molecular Solar Cells (DSC and Polymer solar cells). The research focuses also on understanding how the formation of electrons and holes as well as the charge separation takes place at the atomic level. From this basic understanding, we learn how we can build new and more stable materials and interfaces that can be used efficiently in a solar cell device.
The understanding is obtained by using the characteristics of the X-ray spectroscopy in terms of element specificity and chemical sensitivity and we are constantly developing these methods of experimenting on functional systems to follow the transformation in time and space. In short, the X-ray spectroscopy is used to investigate where the absorption process occurs, where are the electrons, what time does it take for the charges to be transported from atom to atom, and loss-mechanisms including conversion of electrical energy to heat.
People
Håkan Rensmo (professor)
Sergey Butorin (researcher)
Bertrand Philippe (researcher)
Gabriel Man (Postdoc)
Dibya Phuyal (PhD student)
Sebastian Svanström (PhD student)
Selected publications:
Valence Level Character in a Mixed Perovskite Material and Determination of the Valence Band Maximum from Photoelectron Spectroscopy: Variation with Photon Energy
Bertrand Philippe, T. Jesper Jacobsson, Juan-Pablo Correa-Baena, Naresh K. Jena, Amitava Banerjee, Sudip Chakraborty, Ute B. Cappel, Rajeev Ahuja, Anders Hagfeldt, Michael Odelius, Håkan Rensmo.
J. Phys. Chem. C, 2017, DOI: 10.1021/acs.jpcc.7b08948.
Partially Reversible Photoinduced Chemical Changes in a Mixed-Ion Perovskite Material for Solar Cells
Ute B. Cappel, Sebastian Svanström, Valeria Lanzilotto, Fredrik O. L. Johansson, Kerttu Aitola, Bertrand Philippe, Erika Giangrisostomi, Ruslan Ovsyannikov, Torsten Leitner, Alexander Föhlisch, Svante Svensson, Nils Mårtensson, Gerrit Boschloo, Andreas Lindblad, Håkan Rensmo.
ACS Appl. Mater. Interfaces, 2017, 9, 34970-34978.
Chemical Distribution of Multiple Cation (Rb+, Cs+, MA+, and FA+) Perovskite Materials by Photoelectron Spectroscopy
Bertrand Philippe, Michael Saliba, Juan-Pablo Correa-Baena, Ute B. Cappel, Silver-Hamill Turren-Cruz, Michael Grätzel, Anders Hagfeldt, Håkan Rensmo.
Chem. Mater, 2017, 29, 3589-3596.
In-Situ Probing of H2O Effects on a Ru-Complex Adsorbed on TiO2 Using Ambient Pressure Photoelectron Spectroscopy
Eriksson SK; Hahlin M; Axnanda S; Crumlin E; Wilks R; Odelius M; Eriksson AIK; Liu Z; Ahlund J; Hagfeldt A; Starr DE; Bar M; Rensmo H; Siegbahn H.
Top Catal. 2016;59(5-7):583-90.
Electronic Structure of TiO2/CH3NH3Pbl3 Perovskite Solar Cell Interfaces
Lindblad R; Bi DQ; Park BW; Oscarsson J; Gorgoi M; Siegbahn H; Odelius M; Johansson EMJ; Rensmo H.
J Phys Chem Lett. 2014;5(4):648-53.
Atomic and Electronic Structures of Interfaces in Dye-Sensitized, Nanostructured Solar Cells
Johansson EMJ; Lindblad R; Siegbahn H; Hagfeldt A; Rensmo H.
ChemPhysChem. 2014;15(6):1006-17.
Research links
We are members of STandUP for energy and the Center for Molecular devices (CMD). The research platforms include strong links to Department of Chemistry Ångström and Department of Engineering at Uppsala University as well as groups doing similar research at KTH and Stockholm University. It consists of monthly meetings with 30-40 researchers and a large set of shared grants (e.g. SSF, STEM, VR), PhD students, postdocs and researchers as well as publications. Internationally our collaborations include University of Cambridge, United Kingdom and École Polytechnique Fédérale de Lausanne (EPFL), Switzerland.
Master and Bachelor projects
We invite students interested in master and bachelor projects. For more details about available projects, please contact Håkan Rensmo.
Batteries
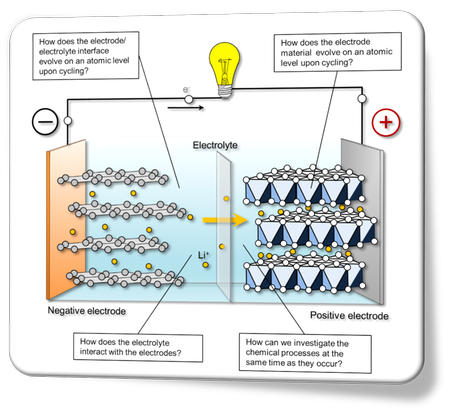
The battery research in our division focuses on applying and developing x-ray based experimental techniques and methodologies for improved understanding of batteries and the reactions occurring during energy conversion. Our research focuses on both the interfaces between the electrodes and the electrolyte as well as on the function of the electrode materials and their evolution during charge and discharge.
A rechargeable battery is an electrochemical device that converts chemical energy into electrical energy and vice versa. The main parts of a battery are the two electrodes and the electrolyte and during battery operation these components may undergo oxidation and reduction reactions, forming new layers at the interfaces. The properties of these layers, especially the layer between the negative electrode and the electrolyte, are of great importance regarding not only battery performances but also battery safety and long-term stability. Information about the electrode materials, e.g. working principle, stability and reversibility is important for the development of new active materials suitable to achieve better batteries.
Most of the research at the X-ray Photon Science is based upon various spectroscopic techniques, and the division has a long track record of method and instrumental development. To investigate energy storage in batteries, photoelectron spectroscopy (link), X-ray absorption (link) and Resonant Inelastic X-ray Scattering (RIXS) (link) are primarily used. The battery research at the division is currently focusing on developing operando x-ray based techniques, where the electrochemical cycling of the battery will be performed in combination with the x-ray based characterisation. In operando battery techniques will primarily be developed for ambient pressure photoelectron spectroscopy (link) and RIXS.
People
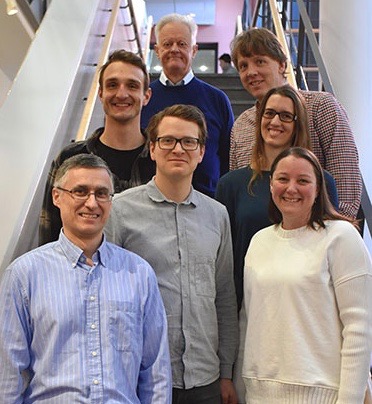
Laurent Duda (Docent)
Maria Hahlin (Assistant Professor)
Ida Källquist (PhD student)
Fredrik Lindgren (Researcher)
Felix Massel (PhD student)
Bertrand Philippe (Researcher)
Håkan Rensmo (Professor)
Hans Siegbahn (Senior Professor)
Selected publications
Examples of published articles from the battery related research at the division.
Oxygen redox chemistry without excess alkali-metal ions in Na2/3[Mg0.28Mn0.72]O2
Urmimala Maitra, Robert A. House, James W. Somerville, Nuria Tapia-Ruiz, Juan G. Lozano, Niccoló Guerrini, Rong Hao, Kun Luo, Liyu Jin, Miguel A. Pérez-Osorio, Felix Massel, David M. Pickup, Silvia Ramos, Xingye Lu, Daniel E. McNally, Alan V. Chadwick, Feliciano Giustino, Thorsten Schmitt, Laurent C. Duda, Matthew R. Roberts, Peter G. Bruce
Nature Chemistry, 2018, DOI: 10.1038/nchem.2923
Breaking Down a Complex System: Interpreting PES Peak Positions for Cycled Li-ion Battery Electrodes
Fredrik Lindgren, David Rehnlund, Ida Källquist, Leif Nyholm, Kristina Edström, Maria Hahlin, Julia Maibach
The Journal of Physical Chemistry C, 2017, DOI: 10.1021/acs.jpcc.7b08923
Anion Redox Chemistry in the Cobalt Free 3d Transition Metal Oxide Intercalation Electrode Li[Li0.2Ni0.2Mn0.6]O2
Kun Luo, Matthew R. Roberts, Niccoló Guerrini, Nuria Tapia-Ruiz, Rong Hao, Felix Massel, David M. Pickup, Silvia Ramos, Yi-Sheng Liu, Jinghua Guo, Alan V. Chadwick, Laurent C. Duda, Peter G. Bruce
Journal of the American Chemical Society, 2016, 138, 11211-11218, DOI: 10.1021/jacs.6b05111
Charge-compensation in 3d-transition-metal-oxide intercalation cathodes through the generation of localized electron holes on oxygen
Kun Luo, Matthew R. Roberts, Rong Hao, Niccoló Guerrini, David M. Pickup, Yi-Sheng Liu, Kristina Edström, Jinghua Guo, Alan V. Chadwick, Laurent C. Duda and Peter G. Bruce
NATURE CHEMISTRY, 2016, 8, 684-691
Electric Potential Gradient at the Buried Interface between Lithium-Ion Battery Electrodes and the SEI Observed Using Photoelectron Spectroscopy
Julia Maibach, Fredrik Lindgren, Kristina Edström, Maria Hahlin
The Journal of Physical Chemistry Letters, 2016, 7, 10, pp 1775-1780, DOI: 10.1021/acs.jpclett.6b00391
Photoelectron Spectroscopy for Lithium Battery Interface Studies
Bertrand Philippe, Maria Hahlin, Kristina Edström, Torbjörn Gustafsson, Hans Siegbahn, Håkan Rensmo
Journal of The Electrochemical Society, 2016, 163 (2), A178-191, DOI: 10.1149/2.0051602jes
A HPXPS experimental method for characterization of solid-liquid interfaces demonstrated with a Li-ion battery system
Julia Maibach, Chao Xu, Susanna K. Eriksson, John Åhlund, Torbjörn Gustafsson, Hans Siegbahn, Håkan Rensmo, Kristina Edström, and Maria Hahlin
Review of Scientific Instruments, 2015, 85, 044101, DOI: 10.1063/1.4916209
Reaction cell for in situ soft x-ray absorption spectroscopy and resonant inelastic x-ray scattering measurements of heterogeneous catalysis up to 1 atm and 250° C
P.T. Kristiansen, T.C.R. Rocha, A. Knop-Gericke, J.H. Guo and L.C. Duda
Review of Scientific Instruments, 2013, 84, 113107
Nanosilicon Electrodes for Lithium-Ion Batteries: Interfacial Mechanisms Studied by Hard and Soft X-ray Photoelectron Spectroscopy
B. Philippe, R. Dedryvère, R. J. Allouche, J. F. Lindgren, M. Gorgoi, H. Rensmo, D. Gonbeau, D, K. Edström
Chemistry of Materials, 2012, 24, 1107
Research links
The division has a strong collaboration with the Department of Chemistry-Ångström and ÅABC, as well as with national and international synchrotron facilities such as MAX IV and ALS. The battery research is also linked to STandUP for energy research platform connecting groups doing energy related research at Uppsala University, Kungliga Tekniska Högskolan and Stockholm University. The division also have independent collaborations with the University of Oxford, Karlsruhe Institute of Technology and are involved in large European projects involving many European battery research teams.
Master and Bachelor projects
We invite students interested in master and bachelor projects. For more details about available projects, please contact Maria Hahlin.
Catalysis
Heterogeneous catalysis and photocatalysis
A catalyst is a material that promotes a certain desired chemical reaction without undergoing a net reaction itself. This is achieved by the catalyst enabling a chemical reaction to proceed along an alternative path that has a lower activation energy than without the catalyst present. The enormous impact of catalysis on society can be illustrated by the fact that about 90% of all commercially produced chemical products in a multi-billion dollar market involve the use of catalysts at some stage of their manufacturing process. A particularly important subtopic is heterogeneous catalysis involving gas/solid or liquid/solid interactions and their interfaces. Linked to this research we are also doing research on functional materials for photocatalysis, i.e. the use of (visible) light for directing chemical reactions.
Traditionally, surface sensitive techniques at ultrahigh vacuum conditions have been used and developed to study and understand the physics and chemistry of catalysts. More recently, x-ray absorption spectroscopy (XAS) and high energy x-ray photoelectron spectroscopy (HAXPES) has been applied for in situ characterization at elevated (yet non-operando) pressure conditions, still leaving a pressure gap to realistic conditions.
Closing the pressure gap
Recently, RIXS has been added to the arsenal of x-ray techniques. RIXS excels particularly at operando studies that involve ambient conditions, which are out of the reach of ordinary electron spectroscopies. RIXS can close this pressure gap, a longstanding issue in the field of heterogeneous catalysis. Two examples of recent studies are (i) ethylene epoxidation over silver where subsurface oxygen species were identified and (ii) in situ formed Co-based catalyst systems for catalytic water splitting. The first study was performed under operando conditions at 1 bar pressure and up to 250° C.
People
Laurent Duda (Docent)
Felix Massel (PhD student)
Bertrand Philippe (Researcher)
Håkan Rensmo (Professor)
Anders Sandell (Professor)
Selected publications:
Reaction cell for in situ soft x-ray absorption spectroscopy and resonant inelastic x-ray scattering measurements of heterogeneous catalysis up to 1 atm and 250° C
Paw Toldbad Kristiansen, TCR Rocha, Axel Knop-Gericke, J.H. Guo, Laurent-C. Duda.
Review of Scientific Instruments, 2013, 84, 113107.
Transition metal doping effects in Co-phosphate catalysts for water splitting studied with XAS
F. Massel, S. Ahmadi, M. Hahlin, Y.S. Liu, J.H. Guo, T. Edvinsson, H. Rensmo, L.-C. Duda.
Journal of Electron Spectroscopy and Related Phenomena.
Nickel-vanadium monolayer double hydroxide for efficient electrochemical water oxidation
Fan K; Chen H; Ji YF; Huang H; Claesson PM; Daniel Q; Philippe B; Rensmo H; Li FS; Luo Y; Sun LC.
Nat Commun. 2016;7:9.
In-Situ Probing of H2O Effects on a Ru-Complex Adsorbed on TiO2 Using Ambient Pressure Photoelectron Spectroscopy
Eriksson SK; Hahlin M; Axnanda S; Crumlin E; Wilks R; Odelius M; Eriksson AIK; Liu Z; Åhlund J; Hagfeldt A; Starr DE; Bär M; Rensmo H; Siegbahn H.
Top Catal. 2016;59(5-7):583-90.
Photochemistry of Carboxylate on TiO2(110) Studied with Synchrotron Radiation Photoelectron Spectroscopy
A. Sandell, D. Ragazzon, A. Schaefer, M. H. Farstad, A. Borg.
Langmuir 32, 11456 (2016).
Master and Bachelor projects
We invite students interested in master and bachelor projects. For more details about available projects, please contact Laurent Duda.
Methodology
Methodology and infrastructure
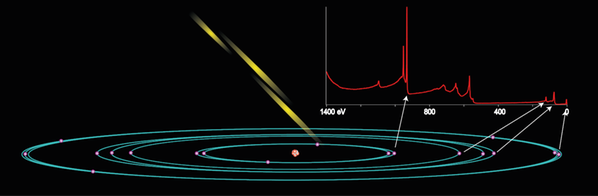
Photoelectron spectroscopy (PES)
Photoelectron spectroscopy (PES) is based on the photoelectric effect and involves measurement of the kinetic energy of electrons that are emitted from a material as a result of their interaction with incident x-ray light. The energy of these photoelectrons gives information on the electronic structure and molecular composition in the sample.
PES is an element specific technique since each element has its own characteristic electronic binding energy distribution of its core level electrons. Smaller adjustment of this binding energy distribution, e.g. chemical shifts, occur due to the interaction with surrounding atoms. Since also the intensity of the emitted photoelectrons correlates with the density of a specific atom it is possible to determine the composition of a sample.
ELEPHANT lab (Ångström laboratory, house 6, floor 0).
SOXPES (Soft X-ray PES) and HAXPES (Hard-X-ray PES)
PES is a surface sensitive technique with a probing depth that can be varied from a few nanometers up to tens of nanometers. The development of synchrotron radiation sources has enabled the use of a wide range of monochromatic photon energies. SOXPES (soft-X-ray photoelectron spectroscopy) and HAXPES (Hard X-ray photoelectron spectroscopy) are two synchrotron-based PES techniques using different photon energy regions, approximately hν < 1500 eV for SOXPES and hν > 2000 eV for HAXPES. The latter is more and more present at synchrotron facilities including beamlines at DESY, BESSY, Diamond and Soleil.
In our research, the modification of the incoming photon energy is a very important tool as it allows us to probe our sample with different probing depth and gives us information over a thickness from a few Ångströms to a few tenths of nanometers. It also allows us to investigate the character or hybridization of the valence states since the cross-section for photoemission depend on the photon energy then depend on the valence level composition.

Panorama of Bessy II.
Ambient Pressure Photoelectron spectroscopy (APPES)
Photoelectron spectroscopy measurements commonly require ultra-high vacuum conditions, which cause volatile substances, e.g. a liquid electrolyte, to be pumped away from the surface of the sample. Recent development of photoelectron spectroscopy instrumentation allows for measurements at higher pressures, so-called ambient pressure photoelectron spectroscopy (APPES), under which pressures a liquid can be stabilized. The division is currently going a step further with the development of the methodology and the instruments to combine the surface analysis and the battery cycling to achieve APPES measurements while the battery is charged or discharged, i.e. going from classical ex-situ post-mortem to in-operando PES measurements on battery materials.
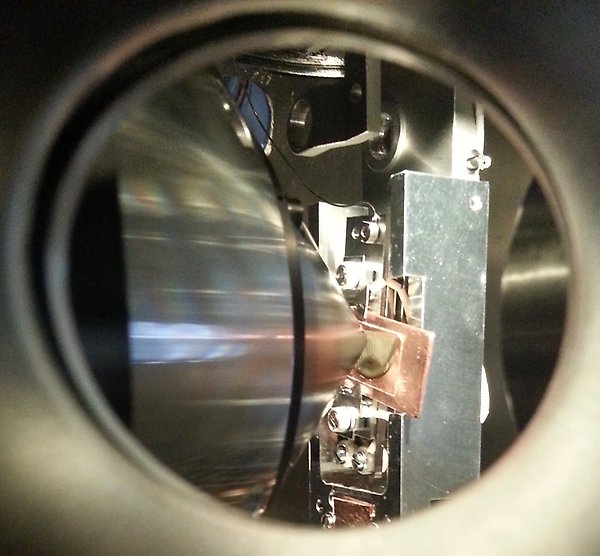
APPES measurement on Si electrode with electrolyte drop.
Resonant inelastic X-ray scattering (RIXS) and X-ray absorption spectroscopy (XAS)
RIXS and XAS are two techniques that provide complementary information about the electronic structure of materials. These are excellent techniques for studying samples in situ and in ambient pressure environments, e.g. Li ion batteries which contain liquid electrolytes and operando catalytic water-splitting studies. XAS usually is typically used to probe the empty states close above the Fermi level of a material. RIXS can either be used to determine neutral excitations in the range between a few meV to several eV or the simply occupied states below the Fermi level of a material. Both techniques have the advantage of providing orbital specific and x-ray polarization sensitive information. A specific new possibility will be the VERITAS beamline at MAXIV.
RIXS has recently experienced a rapid development attracting an ever-increasing number of users and resulting in a quickly rising number of high-profile publications. This development has been made possible by the availability of brilliant synchrotron radiation at new facilities around the world. We perform XAS and RIXS at leading synchrotron facilities and beamlines worldwide, e.g. ADRESS@SLS and BL8@ALS. The X-ray Photon Science division also has a decades-long track record of developing high resolution grating spectrometers for cutting edge research using RIXS. A new advanced x-ray spectrometer has been developed to match the unique capabilities and ultra-high resolution of VERITAS at MAX IV laboratory in Lund and is currently being implemented there.
We have developed, and are continuously improving, sample manipulation platforms containing reaction cells for in situ and operando x-ray studies. These are sample cells that allow flow-through of liquids and gases past an x-ray transparent membrane window. If desired, a solid film may be deposited on the membrane that interacts with the fluid. Examples include the study of atmospheric corrosion, control of water content in gels, heterogeneous catalysis and Li-ion battery cathodes in contact with a liquid electrolyte.
Energy material research at HELIOS
Functionality of the modern devices used in the energy applications often depends on the behaviour of the non-equilibrium charge carriers (e.g. photoexcited electron-hole pairs or conduction electrons). In order to study this, the pump-probe time resolved PES (tr-PES) can be used. One of the beamlines in our in-house HELIOS HHG laboratory is specifically designed for the time-resolved pump-probe spectroscopy and allows to perform angle-resolved pump-probe photoelectron spectroscopy measurements with the overall time resolution better than 50 fs.
HELIOS HHG source produces ultra-short photon pulses (< 35 fs) and covers the energy range from 20 eV to 72 eV, which is ideal to study the valence states of molecules/solids and shallow core levels. The pump photons with the wavelength of 800 nm and 400 nm are currently available. The experimental station is equipped with the modern Angular resolved Time-Of-Flight spectrometer (ArTOF 2) from Scienta Omicron. The high electron transmission of the ArTOF spectrometer (compared to the standard hemispherical electron analyser) allows to minimize the number of photons per pulse required to achieve the good statistics in the photoemission spectra. Moreover, the high angular acceptance of the spectrometer allows to perform “single-shot” Angular Resolved PES (ARPES) measurements while covering the reasonably big fraction of the k-space in both kx and ky directions.
First experiments [1,2] have demonstrated high efficiency of the setup and its advantages compared to the analogous instruments available at the synchrotron radiation facilities. Among them are the very good time resolution (tens of -fs in compare to tens of -ps at the synchrotron), low signal to noise ratio (due to the absence of the higher diffraction orders in the HHG light), stability of the pump-probe synchronization (the same laser is used to produce pump and probe photons).
[1] U. B. Cappel et al., Phys. Chem. Chem. Phys. 18, 21921 (2016)
[2] J. Terschlüsen, Doctoral Thesis, Uppsala University, 2016 (full-text available)
Energy material research at UBjL
At the Infrastructure Uppsala-Berlin joint Laboratory our division is engaged in the build up and running of two beamlines. Both beamlines employ time-of-flight electron spectrometers that have larger transmission at the same resolution as a conventional hemispherical electron analyser. The beamlines exploit this in different ways: the LowDosePES (a) beamline is situated at a dipole source which, together with an x-ray chopper, allow for x-ray radiation sensitive materials to be studied; the second beamline, CoESCA, have two ArTOF spectrometers with the goal of using coincidence spectroscopies. The LowDosePES beamline is equipped with a picosecond laser system that together with the time-structure of the synchrotron X-rays allow us to use pump-probe techniques to study excited electronic states and phase transitions on a picosecond timescale (b). Some examples of our activities is to follow how electrons and atoms move upon visible illumination in materials such as perovskites and polymers.
(a) Erika Giangrisostomi, et al. Low Dose Photoelectron Spectroscopy at BESSY II: Electronic structure of matter in its native state.
Journal of Electron Spectroscopy and Related Phenomena (2017)
(b) Ute B. Cappel, et al. Partially Reversible Photoinduced Chemical Changes in a Mixed-Ion Perovskite Material for Solar Cells.
ACS applied materials & interfaces, 9.40: 34970-34978 (2017)
Ultrafast Magnetization
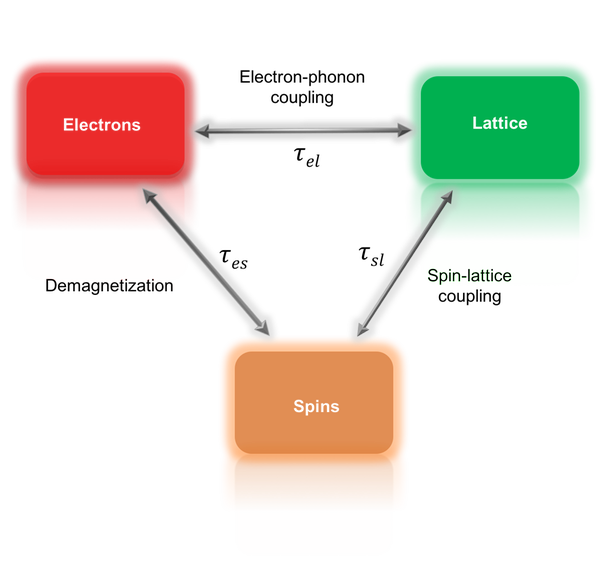
Ultrafast magnetization dynamics
Ultrashort laser pulses can change the magnetization in a material within femtoseconds. The mechanisms that are involved in this demagnetization process have been studied for two decades, since its first observation, but are still under debate. To understand this phenomenon it is necessary to investigate the interactions between the lattice, electron, and spin systems as illustrated by the top figure. Today, two applications in this field that have gained a lot of attention are all-optical-switching and ultrafast generation of spin currents. In general, the investigation of ultrafast magnetization dynamics requires a probe that has femtosecond time resolution. At Helios we use ultra short XUV pulses as the probe, since the high photon energies provide us with elementally resolved information about the magnetization dynamics. This enables us to study how different magnetic components in a compound react to the demagnetizing laser pulse, as well as their subsequent interaction with each other. We use this method to study layered magnetic structures, transition metal oxides, and alloys (ordered and disordered), where we in all cases are interested in studying materials that benefit from the elemental specificity of our source.
T-Moke using XUV
Linearly polarized (p-polarized) light that is reflected by a magnetic sample, changes its reflectivity when the sample magnetization changes. The effect is particularly strong near absorption edges of magnetic elements (changes as large as 50% can be observed). XUV provides the possibility to measure the reflectivity at a specific absorption edge, resulting in elemental specificity. Unlike other magneto-optical Kerr effects, the transverse magneto-optical Kerr effect (T-Moke) has the advantage that no polarization analysis (which is difficult in the XUV regime) is necessary, only the reflected intensity is measured. Furthermore, we are currently investigating the use of circularly polarized XUV pulses for XMCD transmission measurements.
People
- Ronny Knut
- Robert Stefanuik
- Rameez Saeed Malik
- Olof Karis
Selected papers:
A setup for element specific magnetization dynamics using the transverse magneto-optic Kerr effect in the energy range of 30-72 eV
Review of Scientific Instruments 88 (3), 033113
Ferromagnetic Resonance
X-ray ferromagnetic resonance
X-ray ferromagnetic resonance (X-FMR) combines two different techniques, x-ray magnetic circular dichroism (XMCD) and ferromagnetic resonance (FMR), for investigating magnetization dynamics.
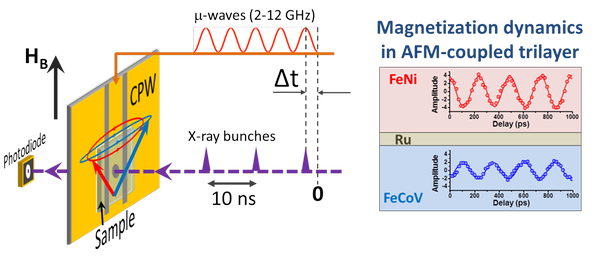
- XMCD: The spin and orbital magnetic moment can separately be quantified by measuring the x-ray absorption at specific absorption edges. Since different elements exhibit energy separated absorption edges in the soft x-ray regime, this technique offers element selective measurements of the magnetization.
- FMR: The magnetic field of microwaves will induce oscillations of the spins in magnetic materials. At certain material specific frequencies, resonances are observed, that exhibit large amplitude oscillations. The frequency dependence of the resonance provides information about effective magnetization, damping, and spin transport.
The combination of XMCD and FMR provides the means to investigate the phase and amplitude of element selective magnetization in complex materials or structures.
These studies are performed at synchrotrons (such as Bessy in Berlin or ALS in Berkley), since they can provide intense circularly polarized soft x-rays with variable energies. The x-ray pulses are synchronized with the microwaves such that the magnetization of each element can be studied at any set phase of the microwave excitation. This is highlighted in the top figure where phase between the top and bottom magnetic layers show an π/2 phase shift due to an antiferromagnetic exchange interaction between the layers. As illustrated by this example, the method provides information about static and dynamic exchange interactions between magnetic elements.
People:
- Somnath Jana (postdoc)
- Yevgen Pogoryelov (Researcher)
- Olof Karis(Professor)
- Ronny Knut(Researcher)
- Rameez Saeed Malik (PhD student)
- Dario Arena (visiting professor)
Papers:
Detection of microwave phase variation in nanometre-scale magnetic heterostructures,
Nature Communications 4, 2025 (2013)
Exploring the accessible frequency range of phase-resolved ferromagnetic resonance detected with x-rays,
J. Applied Physics 113, 033904 (2013)
Spin Transport
Nanoelectronics and spintronics of quantum materials and devices
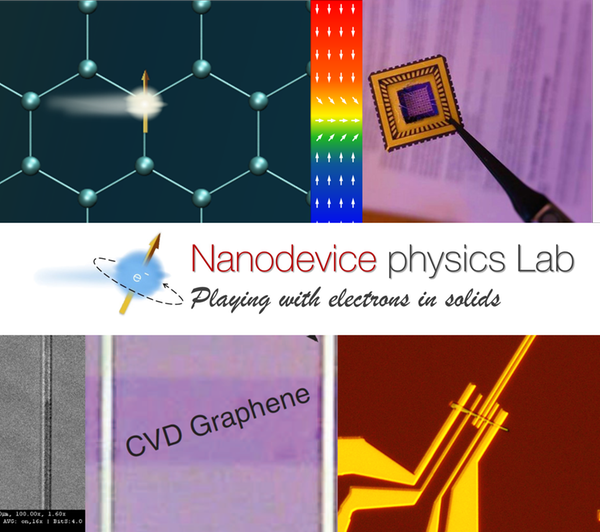
We explore charge and spin transport phenomena in quantum materials and devices. In addition to charge, electrons possess a magnetic property called ‘spin’ that can be Up or Down. Spin-polarized electrons or spins form the basis for spintronics that enabled ultrahigh-density magnetic memory storage and precision sensing, thereby revolutionizing the information technology age. Over the past decade, the advent of two-dimensional materials brought a fresh stimulus for spintronics. For example, Graphene, the first isolated 2D material is ideal for spin transport over length scales of the order of microns at room temperature. We explore graphene circuits to uncover spin relaxation physics and create new device architectures for demonstrating spin functionalities. Beyond graphene, there are 2D semiconductors such as dichalcogenides (MoS2 and MoSe2) and black phosphorus, 2D insulators (hexagonal boron nitride), layered topological insulators (Bi2Se3), the newly discovered 2D magnetic systems (such as Cr2Ge2Te6), all of which provide new opportunities for investigations. Apart from individual crystals, we assemble 2D materials with contrasting properties to prepare special laminates that show improved properties for spin transport with enhanced mobility. In addition to transport based investigations, we study these systems through complementary experimental techniques and computational methods. Our overall goal is to address burning issues in the community and go beyond state of the art to report new physics and device structures for enhanced performance, and realize novel sensing and energy generation applications.
Open position in nanodevice physics and flexible spintronics.
People:
- M. Venkata Kamalakar(Assistant Professor)
- Giuseppe Muscas (Postdoc)
- Ismael Garcia Serrano (Postdoc)
- Jaganandha Panda (Postdoc)
- Fernand Denoel (Master student)
Correlated Materials
Correlated electron systems
Most of the materials used in today’s technologies have properties that are relatively unaffected by electron interactions. Aluminum, diamond or even graphene are materials that may be reasonably explained within the non-interacting electrons model. However, when the interactions between electrons, spins, charges and orbitals become important, novel and fascinating physical phenomena emerge. Such materials are usually named correlated electron systems, and their understanding and possible implementation in future devices, are one of the great challenges of condensed matter physics.
Our interest is to understand why and how unconventional phenomena arise, and how they can be optimized for technological applications. We are studying various types of correlated systems ranging from metal-to-insulator transition, superconductors, topological insulator or magnetic materials. We are investigating their electronic and magnetic properties using a combination of X-ray, neutron and muon techniques at large scale facilities all over the world. In principal, the electronic properties are studied using angle-resolved photoelectron spectroscopy (ARPES) and resonant X-ray scattering (RIXS), while the magnetic properties are probed with elastic/inelastic neutron scattering (ENS/INS) and muon spin rotation (µ+SR). We also synthesize organometallic compounds and thin-film deposition to study quantum magnetism and interface effects. Our experimental results are combined with theoretical models performed in collaboration with the Materials Theory group in Uppsala University and Nordita.
People
- Yasmine Sassa (assistant professor)
- Deepak John Mukkattukavil (PhD student)
- Konstantinos Papadopoulos (Master student)
Selected Publications
Direct observation of orbital hybridisation in a cuprate superconductor
C.E. Matt, D. Sutter, A. M. Cook, Y. Sassa, M. Månsson, O. Tjernberg, L. Das, M. Shi, V. Strocov, K. Hauser, S. Pyon, T. Takayama, H. Takagi, O. J. Lipscombe, S. M. Hayden, T. Kurosawa, N. Momono, M. Oda, T. Neupert, and J. Chang
Nature Communications 9, 972 (2018)
µ+SR Investigation of the Shastry-Sutherland Compound SrCu2(BO3)2
Y. Sassa, S. Wang, J. Sugiyama, A. Amato, H.M. Ronnow, C. Rüegg, and M. Månsson
J. Phys. Soc. Japan 21, 011010 (2018)
The Metallic Quasi-1D Spin-density-Wave Compound NaV2O4 Studied by Angle-Resolved Photoelectron Spectroscopy
Y. Sassa, M. Månsson, O. K. Forslund, O. Tjernberg, V. Pomjakushin, O. Ofer, E. J. Ansaldo, J. H. Brewer, I. Umegaki, Y. Higuchi, Y. Ikedo, H. Nozaki, M. Harada, I. Watanabe, H. Sakurai, and J. Sugiyama
Journal of Electron Spectroscopy and Related Phenomena 224, 79 (2018)
Hallmarks of Hund's coupling in the Mott insulator Ca2RuO4
D. Sutter, C.G. Fatuzzo, S. Moser, M. Kim, R. Fittipaldi, A. Vecchione, V. Granata, Y. Sassa, F. Cossalter, G. Gatti, M. Grioni, H.M. Ronnow, N.C. Plumb, C.E. Matt, M. Shi, M. Hoesch, T.K. Kim, T.R. Chang, H.T. Jeng, C. Jozwiak, A. Bostwick, E. Rotenberg, A. Georges, T. Neupert, J. Chang
Nature Communications 8, 15176 (2017)
Probing two- and three-dimensional electrons in MgB2 with soft x-ray angle-resolved photoemission
Y. Sassa, M. Månsson, M. Kobayashi, O. Götberg, V. N. Strocov, T. Schmitt, N. D. Zhigadlo, O. Tjernberg, and B. Batlogg
Physical Review B 91, 045114 (2015)
Organic Spintronics
Spintronic or spin electronic materials do exploit not only the electron charge but also the electron spin in electron transport applications. They allow to obtain increased functionalities and reduce energy consumption. Molecular or organic spintronics is aiming to exploit the flexibility offered by organic chemistry to obtain new spintronic materials and new applications. This is a field situated between organic chemistry and traditional spintronics. A new development in this area is the use of chiral molecular layers with which as we also have shown, it is possible to produce a new family of spin filter materials. Both long chiral molecular chains can be used as well as small chiral molecules for electron spin filtering purposes. We use, among others, core level spectroscopies for the characterization of organic spintronic materials. The study of core levels in organic compounds allows for the characterization of the electronic, magnetic and real space structure.
Development of new functional magnetic materials
This project uses core level spectroscopy and spectro-microscopy, to study the electronic and real space structure of low dimensional magnetic materials, useful in the context of spin electronics. Together with collaborators from the Universidad Autonoma de Madrid, the Institute for Advanced Studies of Madrid (IMDEA) Nanoscience, and the Institute of Physics of the Polish Academy of Sciences (IFPAN, Warsaw), we have studied at MAX IV-lab, self organized arrays of nanomagnets, ferromagnetic ultra-thin films and chiral molecules on surfaces. In the area of magnetic semiconductors we collaborate with the IFPAN (Warsaw, Poland), and the QSRC center (Seoul, Korea). We characterize the magnetism and electronic structure of magnetically doped ZnO and VO2 in an elemental and electron shell specific basis, focusing on the role of the spin orbit energy in stabilizing the magnetic response upon doping the semiconductor matrix. We use chiral molecule adsorption on ferromagnetic and non-ferromagnetic surfaces to link the induced electron spin polarization with the chiral symmetry.
Characterisation using x-rays at synchrotron radiation facilities
Part of the work is performed at synchrotron radiation laboratories, in particular at the MAX IV laboratory in Lund, Sweden. We study element specific magnetometry, using dichroism in the X-ray Absorption Spectroscopy (XAS) mode, or the reflected and scattered x-rays, after x-ray excitation. X-ray Photoemission Spectroscopy (XPS) and UV Spin Resolved Photoemission Spectroscopy are used if the systems under consideration allow for their application. We perform a spin polarization analysis of the emitted photoelectrons. X-ray Magnetic Circular and Linear Magnetic Dichroism (XMCD and XLMD), X-ray Resonant Magnetic Scattering (XRMS), Extended X-ray Absorption Fine Structure (EXAFS), Magnetic EXAFS (MEXAFS) are used, to probe the local crystallographic and magnetic structure. The high brilliance of the new SPECIES and VERITAS beamlines at MAX IV-lab serves as a strong motivation for this project to use Resonant Inelastic X-ray Scattering (RIXS) at MAX IV-lab, given the high energy resolution the new RIXS spectrometers do achieve at MAX IV-lab. Both the electronic structure and low energy excitations are investigated using RIXS.
Papers
Towards a new class of heavy ion doped magnetic semiconductors for room temperature applications,
Lee, N. G. Subramaniam, I.A. Kowalik et al. Scientific Reports 5, 17053 (2015)
Enantiospecific Spin Polarization of Electrons Photoemitted Through Layers of Homochiral Organic Molecules
Nino, I. Kowalik, F. Luque et al. Advanced Materials 26, 7474 (2014)
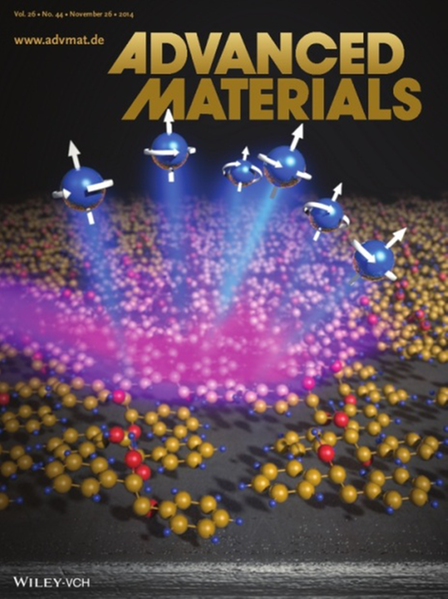
Energy and Environment
Environmental and energy applications
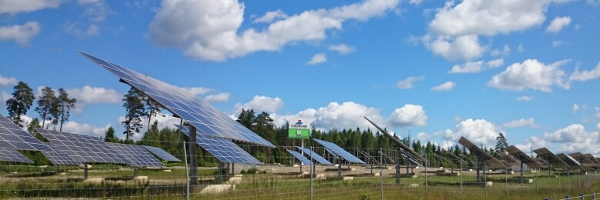
Some of the grand challenges facing humankind are connected to energy and environment. We aim to develop a fundamental understanding of key processes on the atomic level connected to energy conversion and storage, as well as their environmental consequences. Much of the research concerns investigations on complex interfaces and our primarily X-ray-based research includes:
- Photocatalysis for fuel production from sunlight.
- Solar cell materials for improved solar energy conversion.
- Battery materials for improved energy storage.
- Aqueous and atmospheric aerosol surfaces for improved climate prediction.
Areas of research
- Heterogeneous catalysis towards energy storage: Atomic level understanding of chemical bond formation.
- Photon-to-electron energy conversion in next generation solar cells: Energy alignment and charge transfer dynamics.
- Li-ion batteries and beyond: Interface characterization for increased understanding and advancement.
- Atmosphere physics
Contact
Publications
- Hirsbrunner, Moritz; Mikheenkova, Anastasiia; Törnblom, Pontus et al., 2023
Contact
- Programme Professor Condensed Matter Physics of Energy Materials
- Håkan Rensmo
- Head of Division X-ray Photon Sciences
- Nicusor Timneanu